New Mars Timeline
One of the serendipitous features of this
model is that it now allows an independent assessment of the
relative ages of various features and phenomenon on Mars. All
previous efforts to date surface features have had to rely on
relative ages, based on crater counts, normalized to cratering
statistics and radiometric ages from the Moon.63 The tidal model
provides the first truly independent means of calibrating, from a
radically different perspective, the geological history of Mars, if
not other bodies in the solar system (see below).
Thus, a new Mars chronology can now be tentatively proposed.
It is
divided into three main periods:
-
the time from solar system
formation to Mars’ capture by Planet V
-
the period of Mars’
existence as a tidally-locked satellite of Planet V
-
the
interval post-Planet V’s destruction to the Present
Period I
The earliest era of Mars’ history – Period I in our
proposed new timescale -- remains the most uncertain and ambiguous.
In terms of the model presented in this paper, one key reason is the
presence of widespread cratering due to the nearby
explosion/collision of Planets K and V. This pattern of
hemispherically devastating impacts, coupled with the massive
fluvial changes to the northern plains that immediately followed,
have all but eliminated records of earlier Mars’ features from which
reliable reconstruction of its history prior to capture would be
possible.
The nature of the collisional/explosive
“Planets K&V Event” also effectively destroyed the ability to use
relative cratering as any reliable estimate of age, on Mars (and
many other satellites and planets). As previously noted, debris from
this incalculable planetary catastrophe would not only have
bombarded Mars, but also would have spread throughout the solar
system, irrevocably changing cratering statistics and any
crater-based age determinations on a host of other worlds.
Despite this major obstacle, there do seem to be some remaining
clues to dating ancient Martian surface features. Recent publication
of MGS evidence of deep and widespread sedimentary layering
indicates a long period of “warm, wet” climate.64 The presence in
some areas of over 1000 evenly spaced rock layers (presumably from
standing water deposition) also implies that these sedimentary
deposits were controlled by cyclic climatological events. And this,
in one model, then implies some kind of ancient, regular, polar
obliquity shifts and resulting periodic increases in atmospheric
density, from changing solar insolation of the Martian poles.65
Since this kind of cyclic obliquity shifting would be prohibited
after Mars’ capture as a tidally locked satellite, it is proposed
here that these conditions only could have occurred when Mars was
freely orbiting the sun as an isolated world. This implies that Mars
enjoyed a considerable period of “warm, wet” climate early in solar
system history, before its capture by Planet V, and before the
internal radioactive energy sources of Mars died. In this new
chronology, Mars long primordial period of isolated, heliocentric
existence -- Period I – ended with the multi-body capture of Mars by
Planet V.
Period II
In this chronology, Period II dates from the “capture
event” itself, to the destruction of Planet V. Surface evidence of
this major phase of Mars’ geological evolution includes,
-
the beginnings of radial crustal
fracturing around the Tharsis uplift
-
the beginnings of and rapid tidal
enlargement of Valles Marineris from one of these equatorial
rifts
-
the despinning of the planet until a
tidal lock of ~24 hours was achieved
-
the beginnings of the lesser
180-degree Arabia Terra uplift, opposite Tharsis, as a direct
consequence of the establishment of tidal lock
-
the eruption of vast quantities of
NO2, CO2 and H2O into the Martian atmosphere as a direct result
of the accelerated uplift of the tidally-distended Tharsis
A significant increase in water
availability, warming temperatures occasioned by an increased
greenhouse process, and bi-modal pooling of this water into two
stable “east/west” oceans, would have marked what might be termed
this “Garden of Eden “ phase of Martian evolution.
If Planet V possessed one or more additional moons, as
Van Flandern
has proposed, their location in the same system as the tidally
captured Mars, would have occasioned an internal Martian heating
similar (though less intense) to that currently seen in the Io/Europa
situation.66
Thus, for as long as Mars was a satellite of Planet V,
internal energy from a tidally disturbed orbital condition, in
addition to its own dying reserves of radioactive elements, would
have kept its core alive, its magnetic field at full strength, and
its atmosphere constantly replenished. This “idyllic” planetary
situation – Period II - would have ended abruptly some 65 MYA, in
the collision of Planets K &V.
Period III
The last phase of Martian history would have begun with
the destruction of Planet V and the release of Mars back into a
solar orbit. With the sudden relaxation of its previous tidal lock
from Planet V, all the waters collected in Mars’ two bi-modal oceans
would have rushed toward the lowest areas again – mainly the
northern plains.
This unprecedented tsunami situation not
only would have scoured vast portions of the planet’s crust from
Tharsis and Arabia and relocated these previous sedimentary layers
as vast mudflows across the north, the rush of waters would have
carved enormous new “outflow channels” in that crust away from
Tharsis and Arabia – exactly as we see.
As they plunged down the Valles
Marineris system and headed north, some of these now
catastrophically released waters would have buried older “outflow
channels,” from the earlier phases of Valles Marineris’ creation
(Figure 26), under kilometers of additional sediments -- also
confirmed by the new MOLA observations.67
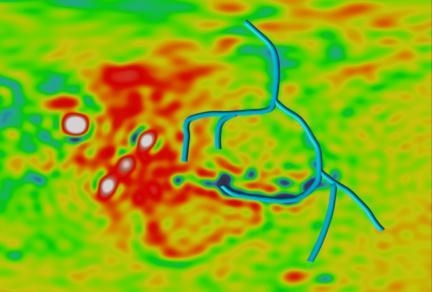
Figure 26
Outflow water
channels beneath and north of Valles Marineris (MOLA)
Recent MGS observations have uncovered additional striking evidence
supporting this “catastrophic collapse” of the former “Tharsis
ocean,” this time northwest of Arsia Mons. Writing in the June 2001
issue of the Journal of Geophysical Research,68 University of
Arizona researcher James Dohm has billed his team’s new findings as
“the largest flood channels in the solar system,” caused by
“catastrophic floods of enormous magnitude” – some 50,000 times the
flow rate of the Amazon.
Located southwest of Olympus Mons
(Figure 27), the newly-discovered channels are 10 times the size of
Kasei Valles, the largest previously known outflow channel system on
Mars. Measuring as wide as 200 kilometers, in our view only the
catastrophic collapse of the former “Tharsis tidal ocean” and the
scouring effect of trillions of tons of newly-released water rushing
north can now account for their existence – exactly as the tidal
model would predict.
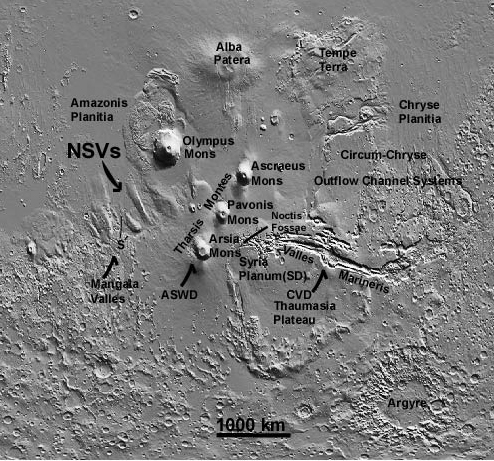
Figure 27
New flood channels on
Tharsis (NVS) --
10 times larger than
any previously discovered, draining northwest.
Strikingly consistent with this model is the bi-modal distribution
of all the Martian “outflow channels.” If the oceans we’ve projected
were bi-modally distributed, as we now state categorically, then the
outflow channels emptying those oceans when the tidal lock collapsed
would also be expected to have a bi-modal distribution in the
geologic record. Again, this is exactly what we see.
Examination of the channel distribution
maps from MGS (Figure 28) reveals that catastrophic outflow channels
draining the two potential tidal oceans are unquestionably also bimodally distributed -- around the periphery of both the Tharsis
and Arabia regions, exactly as the tidal model would predict.
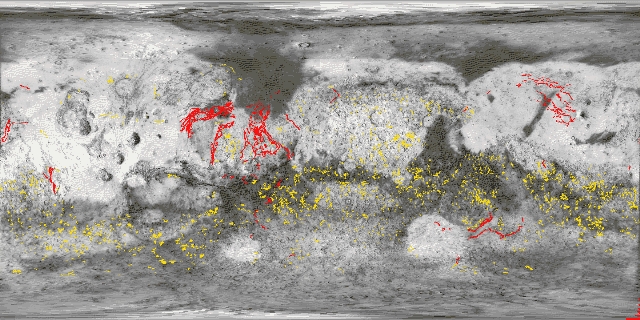
Figure 28
Image map showing
bi-modal outflow channel distribution from Tharsis and Arabia ocean
beds
Apart from the immediate (and catastrophic) relocation of Mars’
oceans, the slow geological relaxation of Tharsis (and to a lesser
extent Arabia) back into the mantle after their partial tidal
support was suddenly removed, would have begun in Period III as
well. This would have created over the following millennia an
inevitable downward warpage of the crust around this massive, now
unsupported uplift, called the “Tharsis trough” (Figure 24). This
inevitable settling would have also triggered additional volcanic
activity both in those regions, and at 90 degrees. The latter we
have now identified with the late creation of Elysium Mons.
The catastrophic arrival on Mars, a few hours after the Planets K&V
collision, of the first debris wave, is marked by the peculiar “line
of dichotomy” of “shoulder-toshoulder” impact cratering that has so
puzzled planetary geologists since 1971. These first impacts would
have begun a long period of Mars continually “mopping up” material
left from the catastrophe near its resultant solar orbit; estimates
for this interval depend on how rapidly the huge quantity of dust
and larger crustal fragments would have either collided with Jupiter
(or other solar system planets), or would have been completely
ejected from the solar system by encounters with these bodies. Those
estimates range from “a few million” to perhaps 100 million years.69
Because of this vast orbital reservoir of condensed core and mantle
materials from the Planets K&V collision, Mars would have
continuously “swept up” new supplies of olivine and iron-rich sulfur
compounds from space for millions of years. This process would have
continually replaced previously fallen materials weathered from
surface exposure to liquid water stemming from irregular periods of
Martian volcanism, triggered by the continuing slow collapse of
Tharsis and Arabia.
In this way, Mars surface history after
the collision ~ 65 MYA – the beginning of Period III -- would have
been a complex tale of episodic “warm, wet” periods in which these
“primitive” materials could be destroyed, followed by cold and arid
intervals in which they could once again accumulate. This episodic
environment likely has extended to the Present, triggered by this
residual internal volcanism from the continued settling of Tharsis,
as well as major obliquity shifts and their periodic warming and
release of current polar reservoirs of CO2 and H2O.
In our model, one direct consequence of this accreted, sulfur-rich
surface environment is the mysterious “stains” themselves. Appearing
initially as extremely dark, flow-like features on sloped surfaces,
it is our proposal that “stains” are created in Period III by
underground liquid water, “wetting” surface sulfur-rich materials.
On the current sands of Mars, composed of iron oxides and trace
sulfur compounds, this would initially produce sulfuric acid.
The acid would then reduce the
iron-sulfur mixtures to an extremely stable, dark black compound --
ferrous sulfide (FeS) -- which would remain visible for years after
this initial “wetting.” 70 Eventually, this black “iron sulfide”
stain would be converted back to reddish iron oxides, via the simple
process of oxidation (Figure 29). This would come from the trace
amounts of free oxygen continuously liberated from Mars’
predominantly carbon dioxide atmosphere by solar ultraviolet
radiation.
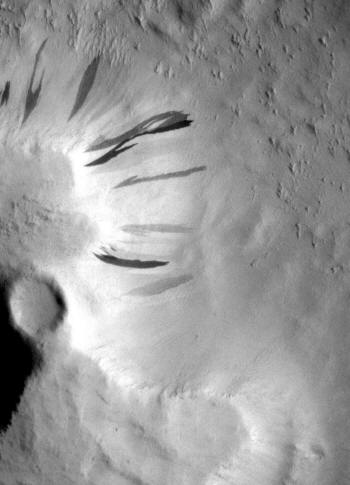
Figure 29
Dark (fresh) flows
alongside lighter faded (older, oxidized) flows (USGS).
Another validation of the tidal model may lie in the recently
published work of Kuzmin R.O. and E.V. Zabalueva Vernadsky. In June,
2000 the two geochemists from the Institute of Geochemistry and
Analytical Chemistry, Russian Academy of Sciences, presented a paper
at NASA’s 31st Lunar and Planetary Science Conference on the
possibility of liquid water on the current Martian surface.
They proposed that the presence of
water-soluble salts in the Martian regolith should influence the
melting temperature of any ice currently trapped in the upper layers
of this icy, porous soil. In the presently observed climate of Mars,
such “icy soil” conditions (they contend) would be expected only
above 40-45 degrees North and South (the ice, in this model, long
having evaporated closer to the Equator, in the billions-of-years
history of Mars). In the Russians’ calculations, the salts’ presence
even in this high-latitude ice-containing-soil could produce a
liquid water phase (seasonally) in a broad range of negative
temperatures.71
In the tidal model, the last major source of liquid water flowed
across Mars only 65MYA -- not “billions.” Therefore, groundwater
would not have had sufficient time to sublimate from the current
Martian soil in regions close to the Equator. In these equatorial
regions, according to our model, now lie the 180-degree seabeds of
two former tidally-locked oceans, whose underlying sediments would
be expected to contain a very high percentage of exactly these
essential water-soluble salts needed to keep subsurface water liquid
under current Martian temperatures.
Thus, the equatorial location of the “stains” (30 degrees plus or
minus), and their clear bi-modal 180-degree distribution, also
strongly suggest – supported by the Russians’ calculations -- that
“stains” do in fact represent current aquifers of liquid, briny
water from those former “twin” Mars’ oceans.
Back to Contents
Implications for Martian Life
It is now undisputed that the
catastrophic events which brought the Cretaceous to a close ~65
million years ago, and resulted in the elimination of the dinosaurs,
also made it possible for mammals to eventually overrun the Earth.
And, some 62 million years later – at the time of the postulated
collision/explosion of the 2nd moon of Planet V, which (according to
Van Flandern) resulted in comets like Hale-Bopp - one of those lines
of mammals was just beginning to assume eventual domination of the
Earth – the primates that would one day lead to us.
These events, we now believe, were inexorably set in motion by the
destruction of Planets K&V.
-
But what of Mars itself?
-
How long did Mars spend as a satellite of
Planet V – in Period II -- before the latter was destroyed?
-
Was
there time enough for life to actually originate upon the planet,
and if so, when – in Period I, before its capture -- or in Period
II, sometime after?
-
If life did evolve on Mars, what can the tidal
theory tell us now about its subsequent development?
-
Are there any
clues contained in the Martian tidal model which could reveal if and
when a “biological capture clock” for Mars was ever started?
As stated earlier, we now know from MGS of the existence of massive,
regular sedimentary layers across major sections of the planet. From
these we can logically infer that Mars experienced a protracted
period of systematic climatological change, most likely in response
to cyclic alterations in its spin axis obliquity – which laid down
sediments in response to these repeating environmental cycles.72
Clearly, this period refers to Mars orbiting the sun as a single
planet -- as such significant obliquity excursions, according to the
work of Lasker et al. (1993) strongly indicate that major
obliquity excursions would have become impossible during Mars’
tenure as a tidally captured satellite.73
Calculations of Mars current isolated obliquity shifts by Wisdom et
al. (1995)74 reveal chaotic excursions up to 60 degrees, and periods
on the order of 3-5 million years. These would inevitably result in
drastic changes in Martian atmospheric density and temperature, as
polar ices melted and refroze – thus easily producing the extensive
sedimentary deposition layers MGS has now discovered.75
Taking one MGS observation “of a
thousand individual layers,” and multiplying by the amount of time
in each potential long-term cycle (~3-5 million years), we arrive at
one estimate of the span of time represented by this pre-capture
phase of Martian evolution: several billion years. This, in effect,
is equal to the ~ 5 billion years since the formation of the solar
system.76 From this we can now estimate that the time Mars spent as
an isolated planet, before capture – in other words, the length of
Period I -- was probably most of solar system history.
So, when did Mars’ capture occur?
Based on extensive new calculations published in recent years, it
appears that “chaotic instability” of the solar system orbital
dynamics can set in even after several billion years.77 These
calculations, however, have been performed without consideration of
two former “missing” planets (this model). It is therefore likely
that our solar system’s stability would be even more chaotic with
their addition to the model – particularly, if (as we and Van Flandern propose) they once inhabited the current region between
Jupiter and Mars. This is due to Jupiter’s disproportionate effect
(from its excessive mass) on all long-term stability considerations.
If basic solar system physics is now questionable, even after
billions of years of apparent “orbital stability,” then it is also
theoretically possible for the “rare” planetary event we’ve proposed
in this paper to have occurred: the close encounter of Planet V with
Mars, with the subsequent ejection of another satellite -- required
to remove sufficient energy for capture. Which again raises the
crucial question: when did this occur?
The capture of Mars by Planet V --through the mechanism of the
ejection of another satellite -- presents a possible independent
means of dating this seminal event. Such an ejected satellite
initially would have assumed its own moderately eccentric orbit of
the sun – resulting in relatively rapid resonance encounters with
either Jupiter or Earth. These close approaches, especially with
Jupiter, would have radically altered its initial heliocentric
orbit, resulting either in eventual complete ejection from the solar
system or eventual catastrophic impact with another planet.
It is our tentative proposal here that such an impact did occur --
with Venus as the target. Because these two events are linked – the
“Mars exchange” of a satellite with Planet V and its eventual Venus
impact – this sequence of events may in fact allow a date as to when
the capture of the planet Mars by Planet V occurred.
Venus is a unique planet. Although often described (because of size
and composition) as a “sister planet to the Earth,” in fact the two
planets could not be more different. From its atmospheric
composition (~ 97% CO2) to its impenetrable clouds of sulfuric acid
(more anomalous surface sulfur …), to its surface temperature (~900
degrees F.), to the pressure at the base of the atmosphere itself
(92 times the Earth’s), Venus’ current environment is as opposite
from the environments of Earth and Mars as one could possible
imagine.
And, unlike the rotational periods of
Earth and Mars and their direction of rotation, Venus spins in the
opposite direction – and takes 243.7 days to make one complete
rotation.78
Magellan spacecraft radar data from its 1990-1994 survey of the
planet revealed a surprising geological discovery: some catastrophic
event appeared to have completely erased the normal range of impact
craters expected from Venus’ earliest eons. The planet appeared,
instead, to have been completely resurfaced in a geologically brief
period via a violent paroxysm of planet-wide volcanism. The
provisional dating of this event: ~500 MYA.79
It is our proposal in this paper that these three phenomena – the
cataclysmic global melting of Venus; the reversal and slowing of its
spin; and the capture of Mars by Planet V - are the result of the
same causal sequence of events: the ejection of a Planet V satellite
at the same time Mars was captured, and the ultimate collision a few
million years later of that massive moon with the second planet from
the sun. This collision not only radically changed the orientation
of Venus’ spin axis to its current retrograde rotation, but the
energy of the event essentially melted the en-tire Venusian surface.
The extremely puzzling anomalous sulfur
abundance seen on Venus (as well as equally disturbing quantities of
argon-40, and even chlorine in the atmosphere – perhaps a signature
of a former Venusian ocean?)80 is thus a direct result, in this
model, of the impact of a major silicate satellite from planet V --
propelled into Venus a few million years after the exchange of Mars
at the inner edge of the (eventual) location of the Asteroid Belt.
This “causal chain,” if we are right, thus dates Mars capture to
~500 MYA.
Remarkably, the major biological event of terrestrial evolution was
occurring coincident with these phenomena: the sudden appearance (in
less than ~40 million years) of all the current advanced life forms
on this planet, called the “Cambrian Explosion.”
81 This was followed
by ~500 million years of subsequent evolution of those life forms,
ultimately resulting in the human species.
If this reconstructed timeline is correct, then the extent of Period
II on Mars – a “warm, wet” capture interval, fed by volcanic
activity stimulated by Mars tidal situation as a satellite of Planet
V --was essentially the same as that for the appearance and
development of advanced life on Earth. This timeline now has
profound implications for the independent evolution of intrinsic
Martian life.
From the evidence we have assembled, we now know that that large
oceans existed during Period II on Mars (otherwise, there would be
no vast flow channels when their tidal lock was suddenly released);
that an atmosphere dense enough to permit a greenhouse effect to
keep that water liquid also had to exist (otherwise, there would
have been no “liquid water” in such vast amounts); and that such an
atmosphere had to have contained (at least toward the end) abundant
amounts of free oxygen (otherwise, the iron currently dispersed
across the Martian surface would not be in its highly oxidized
condition).
These observations all parallel the
simultaneous development of an equivalent environment suitable for
the evolution of advanced life forms on Earth: time, temperature,
liquid oceans, and an oxygen-rich atmosphere.
It is thus our tentative conclusion, based on the model presented
here, that the tidal epoch of Mars – Period II -- may have led
directly to a separate, spectacular evolution of indigenous Martian
organisms. In fact, there is nothing in this data to preclude the
ultimate appearance of intelligence itself.
We only have to be willing to seriously look.
Back to Contents
Predictions
The “Mars tidal model” we’ve presented offers a host
of future, short and long-term observations by which to judge the
full potential of the theory.
In October, 2001, NASA’s next unmanned Mars mission – 2001: Mars
Odyssey – arrives. By December, it will have been aero-braked into
its final, polar orbit and begun a set of unprecedented surface
observations.82 Some of these will be directly related to the
viability of the Martian tidal model we’ve presented.
Odyssey carries three new scientific instruments to Mars: THEMIS, a
combined visual/infrared camera; GRS, a gamma ray spectrometer; and
MARIE, the Mars Radiation Environment Experiment.83 GRS is an
instrument designed to detect gamma ray emission and neutrons via
cosmic ray-excited stimulation from 20 primary elements – including
silicon, oxygen, iron, magnesium, potassium, aluminum, calcium,
sulfur, and carbon.
It is this GRS instrument which will furnish the first definitive
test of the Mars tidal model presented in this paper.
One of the elements GRS will detect is hydrogen. Hydrogen makes up
two thirds of every water molecule. Thus, Odyssey will map for the
first time (to a depth of approximately one meter) the global
distribution of hydrogen on Mars, from which a global distribution
of all subsurface ice and/or liquid water will be inferred.84
The Mars tidal model specifically predicts, based on the currently
observed bi-modal stain distribution in the MOC images, that
Odyssey’s GRS will confirm a superimposed bi-modal distribution of
subsurface hydrogen over Tharsis and Arabia on Mars. From this, a
similar bi-modal distribution of ice and water on the planet will be
inferred. Only the tidal model can properly account for this
unexpected (to all other Mars models) expected global distribution.
But, the Odyssey observations have the potential to confirm a good
deal more.
That Planet V had to be destroyed, thus releasing Mars from its
previous tidal lock configuration, is a given of this model. But, if
the destruction of Planet V and the release of Mars was not via a
collision of the two major planetary bodies (K&V), then the only
viable alternative is a genuine explosion.
One critical test of this hypothesis will present itself via
additional impending Mars Odyssey/GRS observations of Mars. If a
major new energy source exists, based on a revolutionary physics
capable of literally destroying worlds, then one side effect of this
should have been the creation of a series of highly radioactive
short-lived elements in the wake of the Planet V Event.
It is strongly implied, based on the
calculated energies required to “explode” a planet that such a
source would of necessity involve nuclear level effects – in which
case the associated isotopes might well mimic those found in similar
catastrophic stellar detonations.85 Because of the relatively recent
time frame for the proposed destruction of Planet V (~65 MYA),
several elements and isotopes from such a massive, anomalous
nucleosynthesis in the vicinity of Mars – if it took place -- should
still exist. These may include the isotopes aluminum 26, lead 107,
iodine 129, plutonium 244 and samarium 146.
With half-lives ranging from a few hundred thousand to 150 million
years, Odyssey’s GRS should be able to detect gamma ray emission
direct from some of these primary “anomalous” isotopes, if they are
present on Mars in significant amounts. Other direct decay
signatures would include neutrons, as well as electrons and
high-energy helium nuclei. The second Odyssey radiation instrument
-- MARIE – should be extremely valuable in corroborating the latter
anomalous “high-energy phenomenon” currently emanating from Mars’
surface, if they are in fact present.
The global distribution of such radioactive isotopes (or their
daughter products) should also, in the model, conform to the
observed TES data on anomalous mineralogical distributions: divided
again by the “line of dichotomy.” If present, most radioactives (or
their products) from the “Planet V Event” should still be found
covering the southern hemisphere – consistent with an explosion, in
this variation of our model. Measurement of the remaining isotopic
distribution, compared to daughter products, should also
unequivocally determine the date of this Event.
Positive detection of such short-lived radioactive elements on Mars
would raise the stakes enormously. For, not only would the specific
tidal model detailed here be resoundingly confirmed, but a
clarification of precisely how Mars former “parent” planet was
destroyed – via a “new physics,” with all its attendant implications
-- would finally be forthcoming.86
Verification of longer term predictions of this model depend on more
aggressive manned and unmanned Mars missions. Example: insitu
measurement of the still occurring slow collapse of Tharsis back
into the mantle, from a network of seismic stations placed at
strategic points across the surface, should confirm the “recent”
date of this event -- ~65 MYA.
The tidal model also contains a cautionary tale for future missions
and investigations. In the current search for Mars’ elusive water
reservoirs, already some investigators (Malin et al. – 1999) – based
on a few high-resolution MGS imaging of very selected regions of the
northern plains87 have rejected the idea of “ancient oceans.”
The absence of wide-spread, long-term
oceanic features along the margins of the northern plains – for
instance, fluvial-eroded scarps -- argues in their presentations
that Mars never supported long-term, liquid, wind agitated waters on
those plains. And, in the current Martian models, if Mars ever had
significant amounts of standing water (“oceans”), they would have
had to occupy those currently-observed (from MOLA), low-lying
northern plains.
But this is precisely opposite what the tidal model argues: that
Mars’ long-term oceans were not centered around these current
low-lying northern areas -- but around Tharsis and Arabia, with a
vast gap of dry land (including portions of the northern plains)
between. Only when the tidal lock with Planet V was broken, do we
contend that a vast amount of water rushed toward these low lying
northern Martian regions. But, such waters would also have quickly
evaporated and/or frozen – leaving no time to etch classic “oceanic
signatures” across those plains.
The warning is quite clear: without the correct Mars’ model, future
missions and investigations run the serious risk of looking in the
wrong locations for the wrong surface features to test the wrong
geologic models.
Likewise, an aggressive effort to locate fossils and/or evidence of
former intelligence on Mars must focus on the correct regions in
this model. Such investigations, if properly conducted, should
ultimately lead to a confirmation of our now ~500 MYA timeline for
Mars’ parallel biological development with Earth – the discovery of
a variety of truly advanced indigenous fossils (some of them quite
large), and/or even artifacts -- only possible if the tidal model is
substantially correct.
Back to Contents
Conclusions
Richard Feynman was once quoted as saying “You know
you’re on the right track with a new idea, if you put in fifteen
cents and get two dollars back.”
The Mars tidal satellite model we’ve presented here is just
such a “two dollar” idea. It for the first time accounts for a
number of baffling, enduring mysteries about the Red Planet, while
at the same time remaining consistent with each new observation --
such as the recent equatorially constrained, bi-polar “dark stain
phenomena,” and MGS observations of “recent” (<100,000 year) ice
deposits near the Martian surface.88
Previously enigmatic Martian surface features are now elegantly and
simply explained by the significant tidal forces to be experienced
in such a captured orbit. These include a unique tidal erosion
mechanism for the largest canyon in the solar system – Valles
Marineris; the presence of two antipodal “bulges” in the mantle and
crust of Mars – Tharsis and Arabia – raised by these major tidal
forces over time; and the otherwise inexplicable bi-modal
distribution of current fluvial signatures known as “stains,” as the
fossil remnants of two former “bi-modal tidal oceans.”
The Mars tidal model also accounts for,
-
the presence of vast surface and
deep, ancient water channels flowing northward from Valles
Marineris, and now Tharsis
-
the vertical scarp encircling
Olympus Mons
-
the otherwise inexplicable height
and volume of the Tharsis volcanic uplift itself; the location
of the Arabia and Elysium uplifts (at 180 and 90 degrees,
respectively), from Tharsis
-
the formation of the Tharsis
“trench”
-
the extreme difference in crustal
thickness between the hemisphere’s above and below the “line of
dichotomy”
-
the dramatic difference in cratering
patterns between these same two hemisphere’s
-
the sudden fluvial excavation of
massive amounts of material from the Arabia Terra rise
It also accounts for the otherwise
inexplicable presence of high levels of iron, iron oxides, sulfur
and olivine on Mars – all major signatures of some kind of external
“collision/explosion event” recently in solar system history.
The authors fully acknowledge that certain secondary aspects of the
tidal model may not be testable as yet. For instance, it may not be
possible to precisely determine what precipitated the destruction of
Planet V. Two possibilities have been suggested here: either, a
devastating collision with another major object also formed in this
general location of the early solar system; or, the outright
explosion, via a literal “new physics,” of Planet V. Either
mechanism results in the return of Mars to a free orbit of the sun
circa 65MYA, as mandated by this model, and leaves vital surface
clues (for follow-on missions, such as Mars Odyssey) as to this
crucial sequence of events.
However, there is sufficient evidence now consistent with this model
to strongly infer the prior existence of a “Planet V.” This is based
on the clear signatures of its effects now visible in the topography
and geology of Mars, as well as other bodies in the solar system.
The lack of a currently verifiable mechanism for Planet V’s
destruction in no way diminishes the wide-ranging implications of
the striking evidence of its demise, nor the quiet surface testimony
of its profound effect upon the body we call “Mars.”
Finally, there is significant evidence that Mars’ environment -- as
a tidally locked satellite for ~500 million years -- created
conditions astonishingly favorable to the evolution of advanced
biology upon the planet. Recent rediscovery of decades-old Viking
data, indicating the presence of microbes in the soil exhibiting a
24.66-hour Martian circadian rhythm, leave wide upon the possibility
of much higher evolution not yet officially discovered. This
includes the now distinct possibility, based on the eerie parallel
of Mars reconstructed 500 MYA of evolution with the Earth’s, of even
former intelligent inhabitants.
It is the opinion of the authors that the evidence of Mars as a
tidal locked satellite is now sufficiently compelling to begin a
major reassessment of our current view of Mars.
Back to Contents
Acknowledgements
The authors would like to acknowledge the following groups and
individuals for their invaluable contributions to this research:
-
The Enterprise Mission Membership,
for their continued invaluable support of this research.
-
Ron Nicks, for important discussions
of the concept.
-
And Jill England and Effrain
Palermo, for the vital statistical analysis on which the
bi-modal "stain" model is based.
Back to Contents
References
-
1 Recent Results From the Viking
Labeled Re-lease Experiment on Mars Gilbert V. Levin and
Patricia Ann Straat, Journal Of Geo-physical Research Vol. 82,
No. 28 September 30, 1977
-
Nussinov, M.D., Chernyak, Y.B. &
Etinger, J.L., Nature 274, 859-861 (1978).
-
Gilbert V. Levin and Ron L. Levin,
Liquid water and life on Mars, Proceedings of SPIE - The
International Society for Optical Engineering, July 20 1998, San
Diego, California
-
Mars Pathfinder Mission Status, Jet
Propulsion Laboratory, NASA, daily website reports, July 9 –
Aug. 1, 1997
-
http://www.spaceref.com/news/viewpr.html?pid=5626
-
http://www.astro.washington.edu/astro150u/Lectures/lcraters.html
-
“Internal Structure and Early
Thermal Evolution of Mars from Mars Global Surveyor,” Zuber, M.
et al., SCIENCE, March 10, 2000, Vol. 287, #5459, 1788-1793.
-
T. Van Flandern (1978), “A former
aster-oidal planet as the origin of comets”, Icarus 36, 51-74.
-
T. Van Flandern (1993; 2nd edition
1999), Dark Matter, Missing Planets and New Com-ets, North
Atlantic Books, Berkeley, 215-236; 178.
-
Hancock, Graham, “The Mars Mystery
-The Secret Connection Between Earth and the Red Planet,” Crown
Books, ISBN 0-609 60086-9
-
Donald W. Patten and Samuel L.
Windsor, “The Scars of Mars,”
-
Philosophical Transactions of the
Royal Society of London Series A, volume 313 (no. 1524), 5-18
(1984)
-
NASA Activities Dec. 1980, vol. 11,
number 12
-
“Evidence for Recent Groundwater
Seep-age and Surface Runoff on Mars,” Michael C. Malin and
Kenneth S. Edgett. Science
-
http://www.mufor.org/ares/mgs/20968comp.jpg
-
Hoagland, R.C., Bara, M.H.,
“Enterprise Mission Investigators Confirm Existence of Present
Day Water on Mars,” July 18th, 2000
-
“A study of Mars Global Surveyor
(MGS) Mars Orbital Camera (MOC) Images Showing Probable Water
Seepages. Are They Dust Slides as NASA Claims or Proof of Water
on Mars?” Efrain Pallermo, Jill England and Harry Moore,
http://www.users.qwest.net/~vtwild/webpage/SeepsPaper.pdf
-
Edgett, K. S., M. C. Malin, R. J.
Sullivan, P. Thomas, and J. Veverka, Dynamic Mars: “New dark
slope streaks observed on annual and decadal time scales,” Lunar
Planet. Sci. XXXI, Abstract No. 1058, Lunar and Planetary
Institute, Houston, Texas, March 2000. (poster presentation)
-
http://exoplanets.org/index.html
-
“The Race to Epsilon Eridani,”
Schilling, G., Sky and Telescope, June 2001
-
http://www.astro.su.se/~pawel/blois/talk_3.2.html
-
http://www.spaceref.com/redirect.ref?url=adsabs.harvard.edu/books/ormo/&id=2854
-
Woolfson, M., Mon. Not. R. Astron.
Soc. 304, 195-198 (1999); “The satellites of Neptune and the
origin of Pluto”, R.S. Harrington and T.C. Van Flandern. Icarus
39, 131-136 (1979)
-
“The Impact origin of the Moon,” by
Roberto Bugiolacchi.
http://geowords.com/histbooknetscape/l17.htm
-
http://yucc.yorku.ca/~troy/io/io.html
-
http://www.btinternet.com/~motorboat/bore.htm
-
Wu, S. S. C.; Garcia, P. A.; Jordan,
R.; Schafer, F. J.; Skiff, B. A. “Topography of the shield
volcano, Olympus Mons on Mars” - Nature (ISSN 0028-0836), vol.
309, May 31, 1984, p. 432-435
-
http://www.sciam.com/1196issue/1196kargel.html
-
“The Snows of Olympus,” Clarke A.
C., W. W. Norton & Co., New York (1995)
-
Zuber, et.-al, “Internal Structure
and Early Thermal Evolution of Mars from Mars Global Surveyor
Topography and Gravity” –Science Mar 10 2000: 1788-1793
-
http://www.astronomy.com/Content/Dynamic/Articles/000/000/000/455bnnzj.asp
-
ibid.
-
http://www.spaceref.com/redirect.ref?url=adsabs.harvard.edu/books/ormo/&id=2854
-
http://www.nature.com/nsu/010628/01062815.html
-
http://www.sitchin.com/asteroid.htm
-
Schultz, P.H. and Lutz, A.B., "Polar
wan-dering on Mars", Icarus 73, 91-141
-
Donald W. Patten and Samuel L.
Windsor, “The Scars of Mars,” pp. 19-21
-
“The Physical and Chemical
Properties and Resource Potentials of Martian Surface Soils,” C.
Stoker et al., in J. Lewis, M, Mathews, and M. Guerreri, eds.,
Resources of Near-Earth Space, University of Arizona, Tucson,
1993
-
“Solar System Evolution: A New
Perspec-tive,” S. R. Taylor, Cambridge University Press, 1992
-
http://speclab.cr.usgs.gov/mars.tes.fig1.html
-
“Record Of Oxygen From Ancient
Atmosphere Seen In Rocks”
http://unisci.com/stories/20003/0804002.htm
-
ibid.
-
http://speclab.cr.usgs.gov/mars.tes.fig1.html
-
“A Global View of Martian Surface
Com-positions from MGS-TES,” J. L. Bandfield, V. E. Hamilton, P.
R. Christensen, SCIENCE, March 3, 2000
-
“Ancient Geodynamics and Global
Scale Hydrology on Mars,” Phillips, R. et al., SCIENCE, March
15, 2001
-
http://www.astronomynow.com/breaking/990430mars/
-
http://bric.postech.ac.kr/science/97now/99_4now/990429a.html
-
http://math.brandonu.ca/MathModeling/1999/Asteroid.htm
-
Apollo 12 Mission Report, pp. 3-10
to 311, 9-39 to 9-40; Victor Cohn, "Moon Quake Caused by Lem
Called 'Unlike Any' on Earth," Washington Post, Nov. 21, 1969;
Gary V . Latham, Maurice Ewing, Frank Press, George Sutton,
James Dorman, Hosio Nakamura, Nafi Toksoz, Ralph Wiggins, and
Robert Kovach, "Passive Seismic Experiment," in Apollo 12
Preliminary Science Re-port, NASA SP-235 (Washington, 1970), pp.
39-53
-
http://208.154.71.60/bcom/eb/article/7/0,5716,28707+1+28254,00.html
-
T. Van Flandern (1993; 2nd edition
1999), Dark Matter, Missing Planets and New Comets, North
Atlantic Books, Berkeley
-
Personal communication with the
authors.
-
http://www.eoascientific.com/prototype/newcampus/space/11/saturn/saturn17.html
-
Alvarez, L. W., Alvarez, W., Asaro,
F., and Michel, H. V., 1980, “Extraterrestrial cause for the
Cretaceous-Tertiary extinction”: Science, v. 208, p. 1095-1108
-
http://set.lanl.gov/programs/Mars/Atmosphere.htm
-
R.O. Pepin, "Meteorites: Evidence of
Martian origins", Nature 317, (1985)
-
“Are the 'Mars Meteorites' Really
from Mars?” MetaResearch Bulletin, VOLUME 5 (1996)
-
SCIENCE 285, 1364-1365 and 1377&1379
-
http://www.enterprisemission.com/europa.html
-
SCIENCE 285, 1364-1365 and 1377&1379
-
http://www.geocities.com/CapeCanaveral/Galaxy/7881/guide/supplement_swg.html
-
http://www7.pair.com/arthur/meteor/archive/archive4/Feb98/temp/msg00188.html
-
http://www.sipe.com/halebopp/chron91.htm
-
T. Van Flandern (1997), “Comet Hale-Bopp
update”, MetaRes.Bull. 6, 29-32: [The author gratefully
acknowledges Richard Hoagland of the Enterprise Mission for this
argument.
-
“Impact and Explosion Cratering,”
Roddy D. J. et al., eds, (1977) Permagon Press, New York
-
“Sedimentary Rocks of Early Mars,”
Malin, M. and Edgett, K. SCIENCE, December 8, 2000
-
“Water on Mars,” Carr, M., Oxford
University Press, New York, 1996
-
See note 20.
-
http://ltpwww.gsfc.nasa.gov/tharsis/internal_paper.html
-
http://www.space.com/scienceastronomy/solarsystem/mars_flood_010802.html
-
McFadden L. A. (1989) in “Asteroids
II” (R. P. Binzel et al., eds.), University of Arizona Press,
Tucson
-
Chemist Mike Castle, personal
communication.
-
http://www.spacedaily.com/news/marswater-science-00a.html
-
op cit “Sedimentary Rocks of Early
Mars,” Malin, M. and Edgett, K. SCIENCE, December 8, 2000
-
J. Laskar, F. Joutel & P. Robutel,
“Stabilization of the Earth's obliquity by the Moon,” Nature,
vol. 361, 1993, pp. 615-17; J. Laskar & P. Robutel, “The chaotic
obliquity of the planets,” ibid., pp. 608-12
-
http://www.siam.org/meetings/archives/ds95/ip5.htm
-
ibid. “Sedimentary Rocks of Early
Mars,” Malin, M. and Edgett, K. SCIENCE, December 8, 2000
-
op cit.: S.R. Taylor, Solar System
Evolu-tion: A New Perspective, Cambridge University Press, 1992
-
Laskar, J. "A numerical experiment
on the chaotic behavior of the Solar System" Na-ture, 338 (16
Mar 1989):237-238. 11b. Laskar, J. "The chaotic motion of the
Solar System: a numerical estimate of the size of the chaotic
zones." Icarus 88 (Dec 1990):266-291. 11c. Laskar, J., Joutel,
F. and Robutel, P. "The chaotic obliquity of the planets" Nature
361 (18 Feb 1993):608-612. 11dc. Laskar, J. Thomas Quinn, and
Scott Tremaine. "Confirmation of resonant structure in the Solar
System". Icarus 95 (Jan 1992):148-152
-
http://nssdc.gsfc.nasa.gov/planetary/factsheet/venusfact.html
-
Phillips et al. (1992), JGR
97:15,92315,948; Schaber et al. (1992) JGR 97:13,25613,301;
Strom et al. (1994) JGR 99:10,89910,926
-
“Venus Revealed,” p. 120, Grinspoon,
D. H., Addison-Wesley, New York, (1996)
-
http://www.id.ucsb.edu/fscf/library/origins/quotes/cambrian.html
-
http://mars.jpl.nasa.gov/odyssey/
-
http://mars.jpl.nasa.gov/odyssey/technology/index.html
-
http://mars.jpl.nasa.gov/odyssey/technology/grs.html
-
http://www.umr.edu/~om/report_to_fcr/report_to_fcr1.htm
-
http://www.enterprisemission.com/hyper1.html
-
“Oceans or seas in the Martian
northern lowlands: High resolution imaging tests of proposed
coastlines,” by M.C. Malin and K.S. Edgett, Geophysical Research
Letters, v. 26, p. 3049-3052, 1999
http://www.msss.com/mars_images/moc/grl_99_shorelines/
-
“Evidence for recent climate change
on Mars from the identification of youthful near-surface ground
ice,” JOHN F. MUSTARD, CHRISTOPHER D. COOPER & MOSES K. RIFKIN
Nature 412, 26 July 2001, 411-414
Back to Contents
|